News
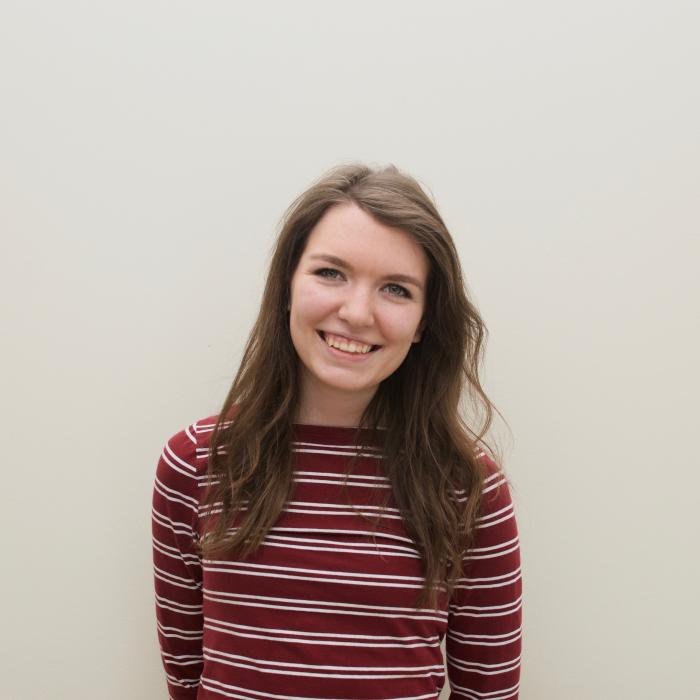
Hannah Musgrove: My Research in the Pompano Group for Five Levels of Understanding
By Hannah Musgrove
UVA ChemSciComm
These summaries describe a research project for five different levels of understanding, going from primary school to expert.
Applied Fabrication Methods for Biomicrofluidic Devices via 3D Printing
Primary School:
When we want to achieve something or learn new things, we need the right tools to do it. For putting a nail in a wall, we usually use hammers. For looking at really small things, like the foot of an ant, microscopes are tools that can help us see. Scientists always need new tools, so having a fast and reliable way to get new tools is important. We look at the best ways to make tiny tools, called “microfluidics,” which let scientists better see tiny living things (like a cell from a person or a plant) under a microscope. We use 3D printing to build tiny microfluidics. 3D printing is a way of making items out of plastic by stacking layers of plastic on top of one another, much like building with Legos. The items we are making with 3D printing must follow three rules to be used as a “microfluidic” tool: 1) it must be very tiny, 2) the tool should be safe for tiny things to live on, and 3) it must be see-through, like glass, so we can see what is on it. We practiced making microfluidic tools this way over and over until they followed all three rules. We are now sharing how we made the tools with other scientists, so they can make their own faster and better than they could before. The faster those tools get made, the faster we can all learn new things by using them!
High School:
In many biological sciences, the ability to learn more about living things on a cellular level is reliant on having the right tools. In order for those tools to work, the materials that make the tools have to follow at least the following rules: 1) be small enough to enable looking at cells and bacteria, 2) be non-toxic so that live cells can live when the tool is being used, and 3) be able to work well with other tools like microscopes, cameras, and lasers so the cells can be seen by the researcher. We also need to be able to make the tools efficiently. For this reason, we are working on methods for 3D printing cell analysis tools called “bio-microfluidics”, which can hold and move living cells within fluid to mimic their natural environment within a body. 3D printing creates these tools by stacking one plastic layer on top of another until an entire plastic device is built. We have practiced using this method with several different kinds of common printing materials to determine the best way to make them as small as possible, low in toxicity, and usable with a microscope. In doing so, we found we could use specified designs to help build small cell-sized features, soak devices in saltwater to cleanse them from toxins, and use nail polish to better see the devices under a microscope. We then developed a guide for creating these tools with this method. Sharing this guide with other researchers in similar fields allows them to also make effective tools quickly so we can all learn more about cells!
College:
Biomicrofluidics are micro-sized devices that allow researchers to better analyze biological samples from human cells to bacteria. These devices could potentially be used in clinical testing for diagnosing disease or specifying treatment. The faster and more effective these devices can be made, the more likely they are to be helpful in such a setting. Thus, 3D printing plastic prototypes is currently considered a promising and efficient method. This method quickly creates mini 3D plastic structures by solidifying liquid resin using light waves, However, there are major obstacles to overcome before biomicrofluidics can be manufactured with this technology. Many of these challenges are due to the composition of the printing resins. During printing, these materials will occasionally not form small enough designs to be useful for making a biomicrofluidic tool. The material can also be toxic to living cells placed on them for analysis, or can be incompatible with the imaging techniques being used. Potential solutions to these problems can be difficult to find, so we have worked to develop a systematic approach to using 3D printing specifically for biomicrofluidic fabrication. We characterized several commercially available printing materials based on their ability to print with high resolution, low toxic impact, and imaging compatibility. We were able to improve on each category using device design techniques, extensive cleaning steps, and polishing methods which enhance the ability to create biomicrofluidics with a 3D printer. x
Graduate Student in the Discipline:
Stereolithographic (SL) 3D printing is a method that utilizes photopolymerization and resin, which can be applied toward microfluidic fabrication. It is currently considered a promising rapid fabrication method for bio-microfluidic applications such as clinical tests, lab-on-a-chip devices, and sensor integrated devices. The benefits of 3D printing led many to believe this fabrication method will accelerate the use of microfluidics, but there are major obstacles to overcome before bioanalytical labs can fully utilize this technology. Most obstacles are due to components within commercially available resins. Challenges include difficulty in producing mechanically stable prototypes with well-defined print resolution, removing cytotoxic components before using with cell culture, and achieving optical compatibility for imaging experiments. Potential solutions to these problems are scattered throughout the literature and are rarely available in head-to-head comparisons. Therefore, we have characterized several commercial 3D printing resins and developed a concise guide for navigating the principles of resin 3D printing that are most relevant for fabrication of bioanalytical microfluidic devices. The fabrication of biomicrofluidic devices via 3D printing was therefore enhanced by improving print resolution via design and choice of resin composition, along with improvements in cytocompatibility and optical transparency of prints via post treatments.
Expert in the Field:
Stereolithographic (SL) 3D printing, especially digital light processing (DLP) printing, is a promising rapid fabrication method for bio-microfluidic applications such as clinical tests, lab-on-a-chip devices, and sensor integrated devices. The benefits of 3D printing led many to believe this fabrication method will accelerate the use of microfluidics, but there are major obstacles to overcome for bioanalytical labs to fully utilize this technology. For commercially available printing materials, this includes challenges in producing mechanically stable prototypes with well-defined print resolution, removing cytotoxic components within many SL resins, and achieving optical compatibility for imaging experiments. Potential solutions to these problems are scattered throughout the literature and rarely available in head-to-head comparisons. Therefore, we have characterized several 3D printing resins and developed a concise guide for navigating the principles of resin 3D printing which are most relevant for fabrication of bioanalytical microfluidic devices. Intended to quickly orient labs that are new to 3D printing, we developed systematic tests to inform resin selection, strategies for design optimization, improvement of biocompatibility via leaching, and enhancement of optical clarity of resin 3D printed bio-microfluidic devices. Overall, the fabrication of biomicrofluidic devices via 3D printing was enhanced to better serve bioanalytical requirements.